Non Wovens by Melt-Blown/Spun Bond Extrusion & their use for Making Masks
Nonwoven fabrics are defined as Web Structures made by bonding or interlocking fibres or filaments by Mechanical, Thermal, Chemical or Solvent means. The predicated growth for the use of synthetic fibres in nonwovens is sufficient guarantee of continued efforts on the part of fibre produces.
Melt Blown Extrusion
This is a manufacturing process that is used for creating a type of fabric called non-woven fabric which is made from polymers such as polypropylene. Traditional fabrics made of natural materials like cotton are woven together, meaning that the material is first formed into a yarn and then interlaced using a weaving or knitting process that results in the creation of a sheet of fabric from the yarn. Non-woven fabrics do not involve joining yarn by weaving or knitting; instead, they mechanically, thermally, or chemically bound together material created from separate fibers of molten polymers forming a web-like fabric. The resulting fabric has a number of desirable properties that include:
- Absorbency.
- Bacterial barrier.
- Cushioning.
- Filtering.
- Flame retardancy.
- Liquid repellency.
- Resilience.
- Softness.
- Sterility.
- Strength.
- Stretch.
- Washability.
Non-woven fabrics are used in a variety of applications, creating products that are used in agricultural, automotive, construction, personal hygiene, roofing, carpeting, upholstery, and medical products, to name just a few examples. Specific examples of the types of products that can be fabricated using non-woven fiber include:
- Filtration, such as HEPA air filters or liquid and gas filter products.
- Masks and respirators for medical and industrial use.
- Disposable medical garbs, such as gowns, drapes, shoe coverings, and head coverings.
- Sanitary products, such as those for feminine hygiene and disposable diapers.
- Oil and liquid adsorbents, which are products that contain spills and pick up oil from the water.
- Coffee filters and tea bags.
- Artificial turf.
- Insulating products.
- Meat and vegetable packing trays.
- Disposable disinfectant wipes.
Here we shall be describing how the melt-blown extrusion process is used to make non-woven fabric, and will then describe how this fabric is then used to create masks such as medical masks, surgical masks and N95 respirators that are critical pieces of personal protection equipment (PPE) used by medical professionals who face exposure on a daily basis to hazardous airborne and aerosolized pathogens.
Melt-Blown Extrusion Process
The melt-blown extrusion process is a single-step process that uses a stream of high-velocity air to blow a molten thermoplastic resin from an extruder die tip onto a conveyor or what is called a take-up screen. The process has been in existence since the 1950s and has grown in significance since its origins. The basic process is illustrated in Figure 1 and is performed using Melt Blown Fabric Extruder Machinery that is specially designed to manage and control the process.
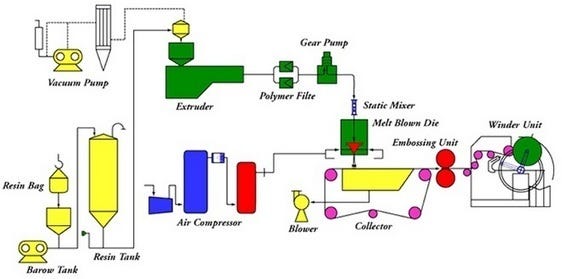
Figure 1 — The components of a typical melt-blown extrusion process.
The basic components of the process are the resin feed system, the extruder assembly, the metering pump, the melt-blown die assembly, the collector, and the winder unit.
Resin Feed System
The raw material for the melt-blown process is a thermoplastic resin in the form of pellets that are stored in a resin bag and gravity-fed to the extruder hopper. There are a number of different polymers that are adaptable for use in melt-blown extrusion. These polymers include:
- Polypropylene [PP]
- Polycarbonate [PC]
- Polybutylene terephthalate [PBT]
- Polyamide [PA]
- Thermo-plastic Polyurathane [TPU]
- Elastic Polypropylene [ePP]
Polypropylene ( PP ) Specifications :
PP granules specially used for producing melt blown non-woven fabric. It is made of high-quality polypropylene as the basic raw material and produced by excellent processing technology. It has stable high fluidity, narrow molecular weight distribution, low ash content, no other product residues, low odor and excellent spinning performance.
Extruder Assembly
The extruder assembly receives the feed of pellets from the resin feed system. A screw impeller similar to an Archimedean screw moves the pellets through a heated barrel of the extruder assembly, where they contact the heated walls and being to melt. There are three zones in the screw impeller — the feed zone, transition zone, and metering zone. The feed zone is the section of the impeller where the material enters the extruder and begins to melt. The transition zone features a decreasing depth and serves to homogenize the polymer feed and compress it. Once the polymer has reached a molten state, it is fed to the metering zone which increases the pressure to prepare the material for discharge through the melt-blown die assembly. At the output of the metering zone of the impeller screw is a screen pack that acts as a filter to trap any dirt or lumps of the polymer from reaching the metering pump.
Metering Pump
The output of molten polymer which is now at 250 oC — 300 oC and pressurized, is fed to the metering pump. The metering pump is a positive displacement pump that is designed to deliver a constant volume of clean polymer mix to the die assembly and accounts for process variations in temperature, pressure, or viscosity of the molten polymer. Within the pump are two intermeshed, counterrotating gears. As the gears rotate, they draw the molten polymer from the intake or suction side of the pump and deliver it to the discharge side of the pump. The metering pump output then feeds to the die assembly.
Melt Blown Die Assembly
Within the die assembly are three key components — the feed distribution, the die nosepiece, and the air manifolds. Two types of feed distribution are commonly used; these are the T-type, which may be tapered or untampered, and the coat hanger type. The coat hanger distribution is more common owing to its even polymer flow.
The die nosepiece is a critical component for determining the uniformity of the resulting web of melt-blown material produced from the machine. The die nosepiece is a tight tolerance wide, hollow, tapered metal part that contains a large number of orifices in it through which the molten polymer will pass to form the melt-blown non-woven fabric.
The air manifolds supply high velocity heated air to the extruded fibers that are outputted from the die nosepiece. An air compressor supplies the pressurized air flow, which is first passed through a heat exchanger drive off a gas or electric furnace to raise the air temperature to a range of between 230 oC — 360 oC at a velocity of between 0.5–0.8 the speed of sound (560–900 feet per second).
Collector
The molten polymer that is extruded through the die nosepiece orifices is then driven by the high-velocity hot air stream from the air manifolds and causes the polymer to form microfibers as they further extend in the air stream (See Figure 2). These microfibers have diameters that range from 0.1 microns to 15 microns. (By comparison, cellulose fibers have a diameter of around 50 microns and a human hair 120 microns.) At the same time the fibers are extending, they are being blown together while in a semi-molten state and directed towards a collector screen. The hot air stream also causes secondary air to be drawn from the surrounding ambient air and helps to cool and solidify the collected web of material that forms on the collector, which is a take-up metal screen attached to a conveyor. The fibers solidify and are randomly laid onto the collector, binding together to form a web by both entanglement and cohesion of fibers to one another. By varying the collector speed and the separation distance between the die nosepiece and the collector, variations in the web fabric density can be achieved to suit different applications. A vacuum pump is often used to draw a vacuum on the inside of the collector screen. This serves to remove the hot air stream and enhances the web-laying process on the collector.
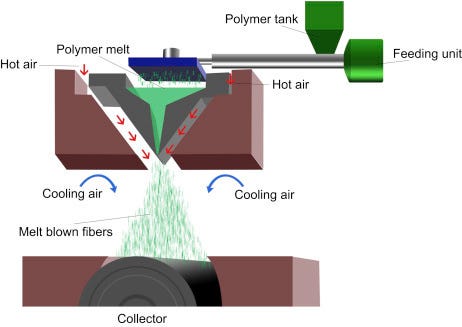
Figure 2 — Polymer fibers forming and being passed to the collector.
Winder Unit
The cooled fabric from the collector is wound onto a cardboard core in the winder unit. For many types of melt-blown non-woven fabrics, there is sufficient cohesion achieved between fibers so that the material is suitable for use without any need for additional bonding. In some applications, further processing of the material may be necessary to alter the material characteristics. Thermal bonding is a commonly used technique when additional bonding is needed, which can increase the material’s strength but with a resulting increase in stiffness and loss of a fabric feel.
After any needed bonding, the production process for melt-blown extrusion of non-woven fabrics is complete. Additional postproduction processes may be applied as needed, such as the addition of flame retardant chemicals, depending on the end-use for the material. The non-woven fabric is then sold to converters who use it as raw material to make filtration products, coffee filters, insulations, or as will be discussed below, medical and surgical masks.
Process variables
The characteristics of the melt-blown non-woven fabric produced can be influenced and controlled to some degree by varying some of the operational conditions and inputs to the process. These include factors such as:
- The type of polymer used and its material characteristics such as molecular weight
- The extruder operating conditions such as temperature
- The geometry of the die nosepiece such as the orifice size and number of orifices
- The hot air stream conditions (temperature, velocity)
- The distance between the die nosepiece and the collector screen
- The speed of the collector
Medical Mask Construction and Mask Making Machinery
Non-woven fabric is a primary material used in the manufacturing of medical and surgical masks. As with the process for non-woven fabric production, specialized Face Mask Production Machinery is utilized to mass-produce large quantities of disposable surgical masks medical masks (See Figure 3). To understand how these machines function, it is necessary to first learn about how these types of masks are constructed.
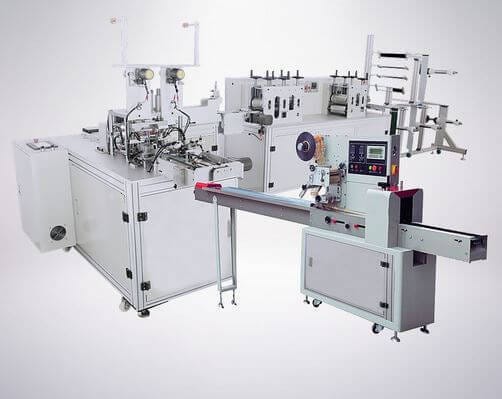
Figure 3 — Automated mask-making machinery
Medical masks typically are created from stacking together three layers of non-woven material. An inner layer that comes in contact with the wearer’s face is used to absorb moisture that is created during normal expiration. An outer layer of non-woven fabric serves as a waterproof barrier that precludes any liquids expelled by the patient while talking, coughing, or sneezing from being transmitted or absorbed by the mask. Sandwiched between the inner and outer layer of the mask is a middle layer that serves as a filter. This middle layer is usually created from polypropylene (PP) melt-blown non-woven fabric and is treated to be an electret. The electret treatment adds electrostatic properties to the filter layer allowing for electrostatic adsorption which helps to trap aerosolized particles via electrostatic attraction.
Mask Making Process
Mask making machinery used to rapidly create disposable medical masks automates the steps needed in the process. The basic process steps for creating flat disposable medical or surgical masks are:
- Combining the three layers of materials together to produce the multilayer mask fabric — The machine takes the different non-woven fabrics from their supports and feeds them together into a layered structure.
- Attachment of the metal nose strip — the machine stitches the flat metal wire onto the 3-layer fabric which will be used by the wearer to fit the mask to their nose and improve its seal to the face.
- Add folds and pleats — the machine uses a folding device to add folds and pleats to the mask that will enable a standard mask to be adjusted to suit different wearers.
- Cutting & stitching — the three-layer material is cut to individual size masks and the edges are stitched to join the layers.
- Attachment of ear loops — ear rope is attached, and adhesive is applied, followed by a thermal press to secure the loops in place. Other methods of attachment include the use of ultrasonic welding.
- Disinfection — medical-grade masks are subjected to a sterilization process using ethylene oxide to render any microbial contamination inactive. Following this treatment, masks must be allowed to stand for a period of 7 days until the ethylene oxide level dissipates, as the material is toxic to the human body as well as being flammable.
- Packaging — following the waiting period, completed masks are packaged for shipment.
Cup-shaped masks and respirators are created using a similar process, but different machinery is employed, and other materials and steps are needed. As an example, the material composition of a 3M™[1] Particulate Respirator Model 8210, which is an N95 type respirator, calls for the use of a polyester shell and coverweb, a polypropylene filter (middle layer), polyurethane nose form, aluminum nose clip, and thermoplastic elastomer straps that secure the respirator for a tight fit to the wearer’s face.
As with the medical mask, the non-woven polypropylene filter layer is key to the filtration performance of the respirator. The random orientation of the fibers from the melt-blown extrusion process that was described earlier combine with the density and fine fiber size to produce a material that can filter out the smallest of particles with high efficiency. These characteristics make the material essential for filtering viruses and other pathogens in medical settings and help explain why non-woven fabrics play a key role in filtration products for various uses.
Face mask production machines are expensive to purchase, representing an investment in the hundreds of thousands of dollars. However, they can produce hundreds of thousands of masks per day with a consistency in quality that makes the investment pay for itself in a short period of time. And in a crisis situation such as the coronavirus pandemic, and automated process is the only way to keep up with the demand for essential PPE such as medical and surgical masks to protect the health of front line workers such as doctors, nurses, and EMTs.
Summary
Here we have provided information on the melt-blown extrusion process for making non-woven fabrics and how that material is used in the production of medical and surgical masks.
Melt Blown Extrusion Sources:
- https://www.inda.org/about-nonwovens/
- https://www.fibre2fashion.com/industry-article/1280/nonwoven-processes-and-applications
- http://blog.fdinonwovens.com/meltblown-nonwoven-fabrics-why-meltblown-applications-will-increase
- https://www.neenah-filtration.com/materials-technologies/synthetic-nonwovens/
- https://www.sciencedirect.com/topics/engineering/melt-blown-process
- https://textilelearner.blogspot.com/2015/04/non-woven-melt-blown-technology.html
- https://textilelearner.blogspot.com/2012/10/melt-blown-polymers-process-flow-chart.html
Mask Making Sources:
- https://www.saintytec.com/surgical-mask-making-machine/
- https://www.sharpertek.com/automated-medical-mask-machine.html
- https://www.testextextile.com/product/medical-face-mask-machine/
- https://www.testextextile.com/fighting-the-coronavirus-top-6-knowledge-to-know-before-investing-in-establishing-disposable-medical-mask-production-lines/
- https://www.yinusainc.com/main/
Spun Bond Extrusion
Spun bonded technique offer superior tensile properties at a weight of fabric while process modifications of filament device, crimp, cross section, degree of bonding etc., can often produce the required property balances needed to meet end use requirements. The simplicity of concept in the manufacture of spun bonded nonwovens is not an adequate reflection of the considerable manufacturing difficulties spun bonding contains as a process.
The products are no longer a novelty to consumers and their acceptance in some areas has been delayed more by the textile industry than by the public at large. This paper is mainly focussed on production, properties and the end use of spun bonded fabrics in Re-engineering manner.
Spun bonding is one of the most popular methods of producing polymer-laid nonwovens. This process is based on the melt spinning technique. The melt is forced by spin pumps through a spinneret having a large number of holes. The quench air ducts, located below the spinneret block, continuously supply the conditioned air to cool the filaments. There is also a continuous supply of auxiliary room temperature air. Over the line’s entire working width, ventilator generated under-pressure sucks the filaments and mixed air down from the spinnerets and cooling chambers.
The through a venture (high velocity low pressure zone) to a distributing chamber, which affects fanning and entanglement of the filaments. Finally, the filaments are deposited as a random web on a moving sieve belt. The randomness is imparted by the turbulence in the air stream, but there is a small bias in the machine direction due to some directionality imparted by the moving belt. The section below the sieve belt enhances the lay down of the filaments. The conveyor belt then carries the spun bonded web to the bonding zone. The web is then bonded either thermally, mechanically or chemically, depending on the material and the desired properties in the final fabric. Thermal point bonding is the most commonly used technique for many applications.
1.1. Some of the main characteristics and properties of spun bonded webs are:
. Random fibrous structure
. Generally the web is white with high opacity per unit area
. Basis weight range between 10 – 200 k/m2
. Fibre diameter range between 15 and 35 �m
. Web thickness range typically 0.2 – 1.5 mm
. High strength to weight ratios compared to other nonwoven, woven and knitted structures.
. High tear strength (for area bonded webs only)
. Planar isotropic properties due to random lay down of the fibres
. Good fray and crease resistance.
Spun bonded webs are finding applications in a variety of end uses. In the early 1970’s spun bond webs were predominately used for durable applications, such as carpet backing, furniture, bedding and geo textiles. By 1980, however disposable applications accounted for an increasingly large percentage, primarily because of the acceptance of lighter spun bonded polypropylene webs as a cover stock for diapers and incontinence devices. The uses of spun bond web can be broadly classified as, automotive, civil engineering, sanitary and medical, packaging, home furnishing, house wrap and roofing.The complex spun bonding process involves many operating variables such polymer throughput, polymer and die temperatures, quench environment, bonding conditions, and material variables such as polymer type, molecular weights molecular weight distribution and many others. All these variables affect the fibre diameter, fibre structure, web lay down, and physical and tensile performance characteristics of spun bond fabrics such as strength, and chemical and thermal resistance are controlled by the characteristics of the polymer systems used. The structure and properties of the final fabric are determined by the polymer and the processing conditions.
2. WEB FORMATION AND BONDING:
The web is formed by the pneumatic deposition of the filament bundles onto a moving belt. The weight of the fabric is determined by the ratio of the fibre formation to belt speed. For the web to achieve maximum uniformity and cover individual filaments must be separated before reaching the belt. This is accomplished by including an electrostatic charge, or mechanical or aerodynamic forces to separate filaments.
Any of the bonding method available, such as chemicals, mechanical and thermal can be used to achieve bonding in this process. Thermal point bonding, which utilizes both temperature and pressure to effect fibre-to-fibre fusion, is the most common method used. To achieve good properties with the retention of optimum hand/feel in the final fabric, it is essential that the surface temperature of the calendar rolls be selected appropriately. Both the strength and elongation increase with bonding temperature and then decreases after an optimal value. The initial increase in the properties is due to good fibre-to fibre bonding with increase in temperature till the optimum. Excessive heat can cause over bonding and alter the material characteristics. The optimum temperature depends on the fibre morphology and the fabric structure. It is evident that in the case of over bonding, the fibre is completely melted in the contact points and in fact spreads beyond the contact point.Observation of the bond areas with the help of a scanning electron microscope yielded important information about the nature of bonding between filaments. The effect of bonding temperature seems to have an overriding effect on the differences in filament properties in determining the nature of the bond. With increase in bonding temperature, the filaments in the bond area gradually lose their round shape and become more flattened. This leads to a greater surface area of the filament participating in the bond to make it more coherent. Increasing the contact time in the calendar nip also causes the filaments to flatten out. The thickness of the bond was about one sixth of the web thickness in most of the cases. Although bond thickness did not change with bonding temperature, the bonding temperature affected the inter fibre fusion and flow of polymer within and out of the bond. There is also change in the structure of the fibres in the bond area as can be seen from the x-ray diffraction pattern before and after bonding.
3. BOND AREA AND SIZE EFFECTS:
The bonding temperature increased the strength and elongation values increased, till the optimum, with webs having higher bond sizes showing higher strength values compared to the webs of smaller bond size. As one can expect, the higher bond area fabrics were Stiffer and had lower breaking elongation, indicating that very high bond area may not be suitable for producing webs suitable for certain applications.4. COMPOSITE STRUCTURES:
Whereas spun bond fabrics are strong, melt blown fabrics are weak, but have very good filtration characteristics. Many of the applications need the balance of these properties. As a result, one of the growing trends is to make composite structures from spun bond and melt blown. These are popularly making composite structures from spun bond and melt blown. These are popularly known as SMS structures, in which thin melt blown layer is sandwiched between two spun bond layers, producing strong fabrics with good barrier properties.
Electro spinning has been gaining a lot of attention these days because of extremely high surface area of the nanofibres. However, the nanofibre webs are very difficult to handle. One of the approaches of taking advantage of barrier properties of nanofibres is to incorporate them with spun bond or melt blown nonwoven webs12. In nanofibres are shown on melt blown web towards left and on spun bond web on the right side. In both the cases, because of the small size, the nano web looks like a film. It is clear that by adding less than 10 percent nanofibres, a large improvement in filtration efficiency of the webs can be accomplished.
5. SPUN – BONDED AND MELT-BLOWN:
The spun-bonding and melt-blowing processes of manufacturing nonwoven have been the fastest growing systems for the past fifteen years, each showing a growth rate of 10-15 % per year. Ever since the commercial production of polyester (Reemay), polypropylene (Typer) and polyethylene (Tyvek) spun-bonds by DuPont, in the early sixties, numerous companies have entered the production of spun-bonded fabrics. The spun-bonding process avoids the process of first converting melt-spun filaments into staple and then carding and bonding them to form fabrics.
The process is shown, when the polymer is extruded through a spinneret and sometimes is drawn by rollers, crimped, and then passed through an air gun (aspirator jet) before being spread on a conveyor belt. To keep the filaments on the conveyor belt a vacuum is pulled through the porous belt. The web thus formed can then be bonded either thermally, be needle punching, by application of latex, or by any other desired system. Sometimes two streams of extruded filaments, one that has a lower melting point than the other, are mixed to achieve thermal bonding. The most commonly used polymers are the thermoplastic type such a polyesters, polypropylene and polyamides.
Tyvek�, which is a trademark of DuPont, is produced by exploding the polymer (polyethylene) that is dissolved in a solvent ad flash freezing the fibrillated polymer on a colleting screen. The process produces a fabric with very fine fibres and the fabrics are bonded by heat. The fabric thus produced is primarily used in protective clothing, packaging, labelling and filtration.
The spun-bonded fabrics are used in a variety of applications, but the bulk of the fabrics are used in diaper linings, civil engineering (geo textiles), and carpet backing applications. Other major uses include : furniture, filtration, bedding, roofing, apparel, medical, packaging, agriculture, coating substrate, electronics, and interlinings. The most commonly used polymer is polypropylene.
6. APPLICATIONS IN GEO TEXTILES:
Nonwoven geo textiles are made of synthetic staple fibres and they are either mechanically and / or chemically bonded. The clear advantages of staple fibre as raw material for nonwoven geo textiles are the greater latitude and flexibility to change the denier and the polymer type. It is also known to yield higher tear resistance under impact load because of their greater extensibility.
6.1. RAW MATERIAL:
Geo textiles made of both synthetic and natural fibres.
6.1.1. POLYPROPYLENE:
. Inertness towards chemicals
. Low specific gravity
. Lower cost of volume ratio
. Easy process ability
6.2. ONLY IN CARTAIN APPLICATIONS NEEDING:7. PRODUCT PROFILE:
7.1. WEIGHT:
Heaviest mass per unit area belongs to a polypropylene fabric of 535 gsm, where as heaviest polyester nonwoven fabric is of 400 gsm.
7.2. APPLICATION:
. Erosion Control
. Separation
. Drainage
. Filtration
.
The lightest fabric is of 180 gsm, a nonwoven made of polypropylene staple fibre.
7.3. THICKNESS:
Nonwoven fabrics are eminently suitable for fluid transportation along the fabric plane. Thickness is one of the important fabric parameter governing this property. Available indigenously made nonwoven geo textiles exhibit thickness ranging between 0.61mm to 5.3 mm at 2 KPa
7.4. STRENGTH:
The strongest nonwoven fabric of 535 gsm and made of polypropylene has a strength of 40 KN/m in the cross machine direction and 24 KN/m in the machine direction. The weakest among the lot has strength of 2.5 KN/m in the machine direction and 4.0 KN/m in the cross machine direction. They are polypropylene nonwoven of 180 gsm.
7.5. ELONGATION:
The nonwovens geotextiles exhibit very high elongation at break ranging from 60 % to 110 % in the machine direction and 40 % to 85 % in the cross machine direction.
A 100 % polypropylene nonwoven fabric of 250gsm reinforced with woven scrims shows comparatively low elongation at break of 40 % in both the machine and the cross machine direction. This fabric is claimed to be suitable for re-inforcement application.
7.6. OTHER PROERTIES:
Typical properties which are important for drainage applications exhibit the following ranges.8. APPLICATION OF SPUN BONDED POLYPROPYLENE IN MEDICAL TEXTILES:
The soft hand and hydrophobic properties make PP nonwovens particularly suitable for hygiene products, baby diapers and adult incontinence products. Spun bond and melt blown are two main processes for polypropylene nonwoven fabrication. Both techniques require PP resins with high melting flow rate and relatively very narrow molecular weight distribution. The fibres produced in spun bonded nonwovens are spun filaments, whose diameters are in the range of 10-35 microns, whereas the fibres of melt blown nonwovens are usually discontinuous and much finer, typically less than 10 microns.
The melting point of polypropylene (160 – 170oC) is an advantage in many nonwovens. PP fibre can be softened sufficiently to bond to one another without destroying fibre properties. Nonwoven fibres made from polypropylene can therefore be fusion bonded, eliminating the need for chemical binders. The benefits of this technique are from both energy saving and environmentally friendliness. Uses of thermally bonded cover stock in baby diapers and similar products will result in markedly increased use of polypropylene. The fusion characteristics of polypropylene are used not only to bond carded webs but also to improve the dimensional stability of needle bonded fabrics.
To increase the volume of insulation of disposable products in order to avoid disinfecting and destroying harmful micro organisms and prevent crossing infection and to reduce the costs of disposable nonwovens. Medical applications consume the second – largest volume of non-woven fabrics, of which the PP portion totals in excess of 105 million 1b/yr. PP is converted to nonwoven textile goods by air attenuation processes that produce very fine, highly oriented fibres and deposits them as a random mat. The spun-bonding and melt-blowing processes are used separately and in combination to produce a wide range of composite structures including surgical and isolation gowns, drapes, central supply room sterilisation wraps, face masks and pharmaceutical filter media, etc. The spun bond layer provides strength to the structure while the melt-blown layer provides barrier properties with good breath ability. The most attractive prospects for growth in this use area will be in the critical hospital applications where reusable woven textiles are not suitable.
9. CONCLUSION
Both the spun bond and the melt blown nonwovens are continuing to grow and increase their market share. Although they have some process similarities the structure and properties of the fibres and fabrics are entirely different. By the combination of material selected and by varying the different processing conditions, it is possible to produce fabrics with a wide range of properties. This helps in engineering fabric structures suitable for a particular application. Many of the processing conditions have to be optimized for different materials and for different fibre morphologies for the same material. In designing useful products, the approach is to combine theses different processes and produce composite fabric structures. This will help in getting better performance in a more economical way.